Life Cycle Assessment of New Closed-Loop Pumped Storage Hydropower Facilities
NREL has developed a tool that enables developers to evaluate the life cycle greenhouse gas emissions associated with new, domestic closed-loop pumped storage hydropower facilities.
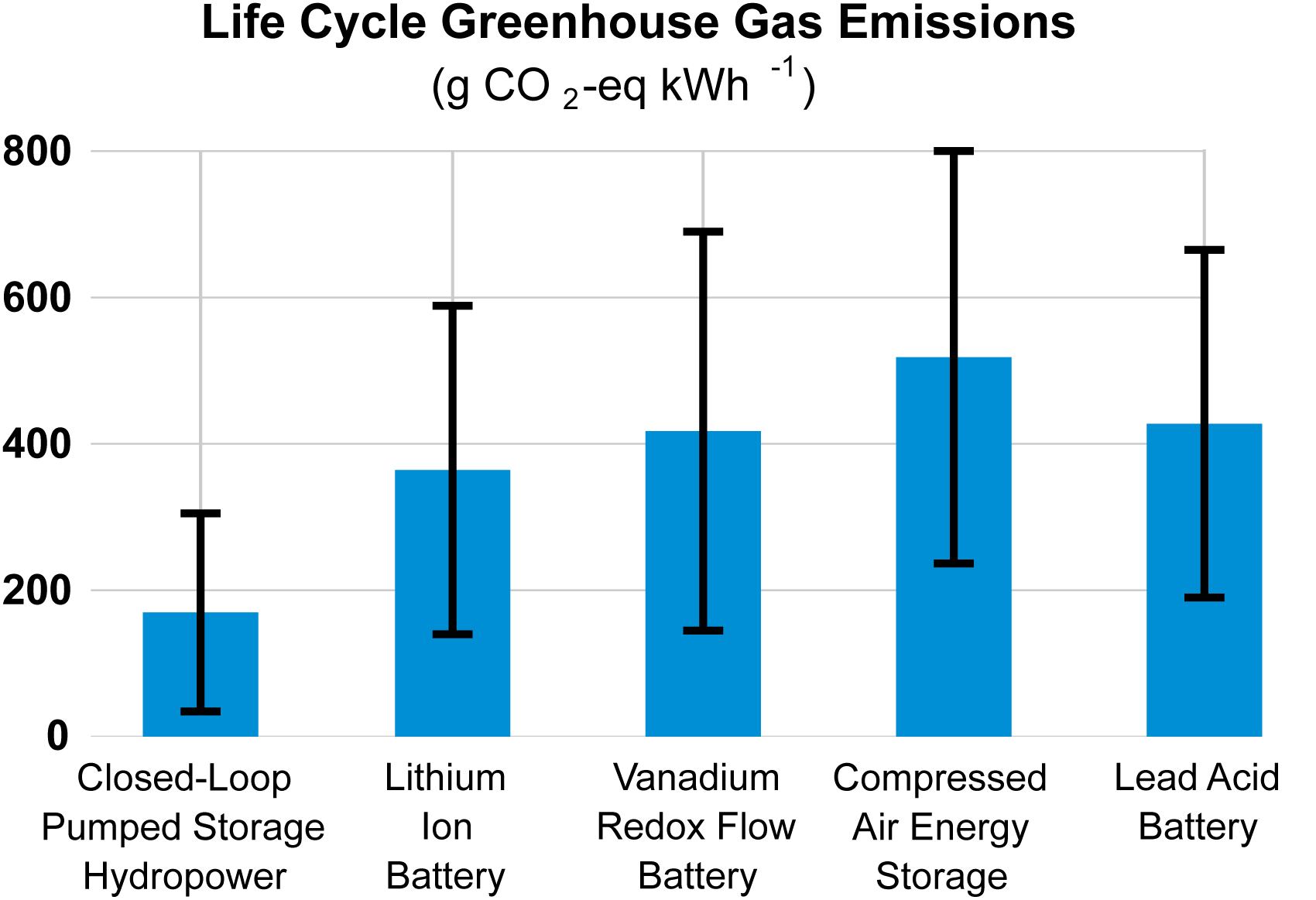
In a 2023 study, NREL researchers compared the life cycle greenhouse gas emissions of closed-loop PSH with other energy storage technologies, finding PSH to have the lowest life cycle emissions among the technologies studied. The black bars represent a range of scenarios explored in the study. Graphic by Tara Smith, NREL
Pumped storage hydropower (PSH) is an established technology that can provide grid-scale energy storage and support an electrical grid powered in part by variable renewable energy sources such as wind and solar. Despite recent interest in PSH, questions remain regarding the overall sustainability of PSH projects, and information about the life cycle of greenhouse gas (GHG) emissions associated with PSH technologies has been limited—until now.
In 2023, NREL researchers published a wide-ranging study that included a full life cycle assessment of new closed-loop PSH projects in development in the United States. The majority of GHG emissions from PSH are attributed to the grid mix of energy used to pump water from a facility’s lower reservoir to its upper one, as this mix is not usually made of 100% carbon-free energy sources. As such, GHG emission levels decrease in locations with a higher level of renewable energy sources in the grid mix. Additional emissions stem from a plant’s construction (e.g., from diesel-powered equipment, concrete, or steel) and ongoing plant operations.
In the study, researchers compared their results to published data on the GHG emissions of other energy storage technologies, including compressed air energy storage and different battery types. The results showed that GHG emissions associated with PSH were lowest among the group studied.
Interactive Data
The success of the study inspired the creation of an interactive tool on OpenEI that uses the study data to enable developers to calculate the GHG emissions of potential PSH sites in the United States—with the goal of promoting PSH development with configurations and locations with the lowest global warming potential.
Users can input specifications for PSH facilities at varying levels of detail, such as reservoir volume, dam material and dimensions, number and capacity of turbines, and the length of the transmission line that connects the PSH system to the grid. They can then compare different PSH scenarios side by side and view the emissions by component, material, and life cycle phase.
Using the Tool
To use the tool, users first select between a Basic and an Advanced scenario, in which they can specify a site configuration and explore GHG outcomes. Basic mode offers a smaller set of options for a simpler user experience, whereas Advanced mode allows the user to submit detailed specifications for PSH system components (e.g., number of reservoirs being built, dam material, and distance to grid connection).
Multiple scenarios with different inputs can then be viewed side by side and subsequently edited with different inputs to produce the desired outcome.
Tool Methodology
The tool was built using the data and methods from the 2023 study, where researchers conducted a life cycle assessment of closed-loop PSH under a variety of assumptions. This data includes all GHG emissions from facility construction, operation, and maintenance and exclude any emissions that might occur during decommissioning or any reservoir-based emissions. We do not consider nonpower uses of the PSH site, which in practice could bear some responsibility for life cycle GHG emissions.
The dataset was built using raw data from proposed PSH sites in the preliminary permitting phase with the Federal Energy Regulatory Commission. For sites with alternative plant design options, the additional configurations are also included in the overall dataset. Each configuration includes an expected annual amount of electricity delivered, which reflects how the plant is expected to operate.
Researchers used methods established in the literature to quantify the GHG emissions of materials and energy inputs in the closed-loop PSH system. A calculation was performed for every configuration (e.g., the GHG emissions per kilogram of concrete for a dam or per kilogram of steel for the powerhouse). Researchers then took a weighted average across the configurations to create a complete inventory of PSH GHG emissions for each material and energy input. View the tool's psh-lca-fact-sheet for further explanations of the calculations.
Guidelines from the Intergovernmental Panel on Climate Change were used in the study to calculate total GHG emissions (kilograms of carbon dioxide equivalent) from individual chemical emissions for each component, material, and life cycle phase as well as in total. Operational emissions associated with electricity used to pump water to the upper reservoir are based on the average emissions intensity of the contiguous U.S. electric sector as determined by NREL's Regional Energy Deployment System grid planning model. In these calculations, all emissions are attributed to the power production function of the PSH plant and do not take into account any other uses of the site.
These methods allow the tool to estimate life cycle GHG emissions across a wide range of specifications for PSH site design and operation, such as alternative electricity mixes, plant lifetime, or plant size. GHG estimates can then be compared with those of other storage and grid technologies to help understand comparative technology tradeoffs.
Publications
Life Cycle Assessment of Closed-Loop Pumped Storage Hydropower in the United States, Environmental Science & Technology (2023)
Life Cycle Assessment for Closed-Loop Pumped Hydropower Energy Storage in the United States, Hydrovision International, NREL Presentation (2022)
Documentation For Material and Energy Input Calculations (2024)
Contact
Share
Last Updated Dec. 11, 2024